Monitoring RNA–Ligand Interactions Using Isothermal Titration Calorimetry
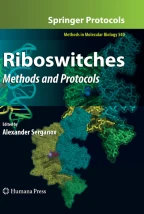
Isothermal titration calorimetry (ITC) is a biophysical technique that measures the heat evolved or absorbed during a reaction to report the enthalpy, entropy, stoichiometry of binding, and equilibrium association constant. A significant advantage of ITC over other methods is that it can be readily applied to almost any RNA–ligand complex without having to label either molecule and can be performed under a broad range of pH, temperature, and ionic concentrations. During our application of ITC to investigate the thermodynamic details of the interaction of a variety of compounds with the purine riboswitch, we have explored and optimized experimental parameters that yield the most useful and reproducible results for RNAs. In this chapter, we detail this method using the titration of an adenine-binding RNA with 2,6-diaminopurine (DAP) as a practical example. Our insights should be generally applicable to observing the interactions of a broad range of molecules with structured RNAs.
This is a preview of subscription content, log in via an institution to check access.
Access this chapter
Subscribe and save
Springer+ Basic
€32.70 /Month
- Get 10 units per month
- Download Article/Chapter or eBook
- 1 Unit = 1 Article or 1 Chapter
- Cancel anytime
Buy Now
Price includes VAT (France)
eBook EUR 85.59 Price includes VAT (France)
Softcover Book EUR 152.96 Price includes VAT (France)
Tax calculation will be finalised at checkout
Purchases are for personal use only
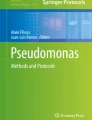
Characterization of Molecular Interactions Using Isothermal Titration Calorimetry
Chapter © 2014
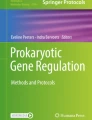
Analysis of Protein–DNA Interactions Using Isothermal Titration Calorimetry: Successes and Failures
Chapter © 2022
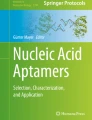
Label-Free Determination of the Dissociation Constant of Small Molecule-Aptamer Interaction by Isothermal Titration Calorimetry
Chapter © 2016
References
- Mizoue, L. S., and Tellinghuisen, J. (2004). Calorimetric vs. van’t Hoff binding enthalpies from isothermal titration calorimetry: Ba 2+ -crown ether complexation. Biophys. Chem. 110, 15–24. ArticlePubMedCASGoogle Scholar
- Leulliot, N., and Varani, G. (2001). Current topics in RNA-protein recognition: control of specificity and biological function through induced fit and conformational capture. Biochemistry 40, 7947–7956. ArticlePubMedCASGoogle Scholar
- Gilbert, S. D., Mediatore, S. J., and Batey, R. T. (2006). Modified pyrimidines specifically bind the purine riboswitch. J. Am. Chem. Soc. 128, 14214–14215. ArticlePubMedCASGoogle Scholar
- Gilbert, S. D., Stoddard, C. D., Wise, S. J., and Batey, R. T. (2006). Thermodynamic and kinetic characterization of ligand binding to the purine riboswitch aptamer domain. J. Mol. Biol. 359, 754–768. ArticlePubMedCASGoogle Scholar
- Wang, L., Kumar, A., Boykin, D. W., Bailly, C., and Wilson, W. D. (2002). Comparative thermodynamics for monomer and dimer sequence-dependent binding of a heterocyclic dication in the DNA minor groove. J. Mol. Biol. 317, 361–374. ArticlePubMedCASGoogle Scholar
- Kaul, M., Barbieri, C. M., Srinivasan, A. R., and Pilch, D. S. (2007). Molecular determinants of antibiotic recognition and resistance by aminoglycoside phosphotransferase (3′)-IIIa: a calorimetric and mutational analysis. J. Mol. Biol. 369, 142–156. ArticlePubMedCASGoogle Scholar
- Batey, R. T., Gilbert, S. D., and Montange, R. K. (2004). Structure of a natural guanine-responsive riboswitch complexed with the metabolite hypoxanthine. Nature432, 411–415. ArticlePubMedCASGoogle Scholar
- Mandal, M., Boese, B., Barrick, J. E., Winkler, W. C., and Breaker, R. R. (2003). Riboswitches control fundamental biochemical pathways in Bacillus subtilis and other bacteria. Cell113, 577–586. ArticlePubMedCASGoogle Scholar
- Feig, A. L. (2007). Applications of isothermal titration calorimetry in RNA biochemistry and biophysics. Biopolymers87, 293–301. ArticlePubMedCASGoogle Scholar
- Kaul, M., Barbieri, C. M., and Pilch, D. S. (2005). Defining the basis for the specificity of aminoglycoside-rRNA recognition: a comparative study of drug binding to the A sites of Escherichia coli and human rRNA. J. Mol. Biol. 346, 119–134. ArticlePubMedCASGoogle Scholar
- Kaul, M., and Pilch, D. S. (2002). Thermodynamics of aminoglycoside-rRNA recognition: the binding of neomycin-class aminoglycosides to the A site of 16S rRNA. Biochemistry41, 7695–7706. ArticlePubMedCASGoogle Scholar
- Pilch, D. S., Kaul, M., Barbieri, C. M., and Kerrigan, J. E. (2003). Thermodynamics of aminoglycoside-rRNA recognition. Biopolymers70, 58–79. ArticlePubMedCASGoogle Scholar
- Gilbert, S. D., Love, C. E., Edwards, A. L., and Batey, R. T. (2007). Mutational analysis of the purine riboswitch aptamer domain. Biochemistry46, 13297–13309. ArticlePubMedCASGoogle Scholar
- Bernacchi, S., Freisz, S., Maechling, C., Spiess, B., Marquet, R., Dumas, P., and Ennifar, E. (2007). Aminoglycoside binding to the HIV-1 RNA dimerization initiation site: thermodynamics and effect on the kissing-loop to duplex conversion. Nucleic Acids Res. 35, 7128–7139. ArticlePubMedCASGoogle Scholar
- Diamond, J. M., Turner, D. H., and Mathews, D. H. (2001). Thermodynamics of three-way multibranch loops in RNA. Biochemistry40, 6971–6981. ArticlePubMedCASGoogle Scholar
- Mikulecky, P. J., Takach, J. C., and Feig, A. L. (2004). Entropy-driven folding of an RNA helical junction: an isothermal titration calorimetric analysis of the hammerhead ribozyme. Biochemistry43, 5870–5881. ArticlePubMedCASGoogle Scholar
- Hammann, C., Cooper, A., and Lilley, D. M. (2001). Thermodynamics of ion-induced RNA folding in the hammerhead ribozyme: an isothermal titration calorimetric study. Biochemistry40, 1423–1429. ArticlePubMedCASGoogle Scholar
- Takach, J. C., Mikulecky, P. J., and Feig, A. L. (2004). Salt-dependent heat capacity changes for RNA duplex formation. J. Am. Chem. Soc. 126, 6530–6531. ArticlePubMedCASGoogle Scholar
- Recht, M. I., and Williamson, J. R. (2001). Central domain assembly: thermodynamics and kinetics of S6 and S18 binding to an S15–RNA complex. J. Mol. Biol. 313, 35–48. ArticlePubMedCASGoogle Scholar
- Recht, M. I., and Williamson, J. R. (2004). RNA tertiary structure and cooperative assembly of a large ribonucleoprotein complex. J. Mol. Biol. 344, 395–407. ArticlePubMedCASGoogle Scholar
- Milligan, J. F., Groebe, D. R., Witherell, G. W., and Uhlenbeck, O. C. (1987). Oligoribonucleotide synthesis using T7 RNA polymerase and synthetic DNA templates. Nucleic Acids Res. 15, 8783–8798. ArticlePubMedCASGoogle Scholar
- Montange, R. K., and Batey, R. T. (2006). Structure of the S-adenosylmethionine riboswitch regulatory mRNA element. Nature441, 1172–1175. ArticlePubMedCASGoogle Scholar
- Gilbert, S. D., Montange, R. K., Stoddard, C. D., and Batey, R. T. (2006). Structural studies of the purine and SAM binding riboswitches. Cold Spring Harb. Symp. Quant. Biol. 71, 259–268. ArticlePubMedCASGoogle Scholar
- Agalarov, S. C., Sridhar Prasad, G., Funke, P. M., Stout, C. D., and Williamson, J. R. (2000). Structure of the S15,S6,S18-rRNA complex: assembly of the 30S ribosome central domain. Science288, 107–113. ArticlePubMedCASGoogle Scholar
- Orr, J. W., Hagerman, P. J., and Williamson, J. R. (1998). Protein and Mg(2+)-induced conformational changes in the S15 binding site of 16S ribosomal RNA. J. Mol. Biol. 275, 453–464. ArticlePubMedCASGoogle Scholar
- Mandal, M., and Breaker, R. R. (2004). Adenine riboswitches and gene activation by disruption of a transcription terminator. Nat. Struct. Mol. Biol. 11, 29–35. ArticlePubMedCASGoogle Scholar
- Turnbull, W. B., and Daranas, A. H. (2003). On the value of c: can low affinity systems be studied by isothermal titration calorimetry? J. Am. Chem. Soc. 125, 14859–14866. ArticlePubMedCASGoogle Scholar
- Tellinghuisen, J. (2008). Isothermal titration calorimetry at very low c. Anal. Biochem. 373, 395–397. ArticlePubMedCASGoogle Scholar
- Tellinghuisen, J. (2005). Optimizing experimental parameters in isothermal titration calorimetry. J. Phys. Chem. B109, 20027–20035. ArticlePubMedCASGoogle Scholar
- Mizoue, L. S., and Tellinghuisen, J. (2004). The role of backlash in the “first injection anomaly” in isothermal titration calorimetry. Anal. Biochem. 326, 125–127. ArticlePubMedCASGoogle Scholar
- MicroCal, LLC. (2003), Northampton, MA. Google Scholar
- Briggner, L. E., and Wadso, I. (1991). Test and calibration processes for microcalorimeters, with special reference to heat conduction instruments used with aqueous systems. J. Biochem. Biophys Methods22, 101–118. ArticlePubMedCASGoogle Scholar
- Liu, Y., and Sturtevant, J. M. (1997). Significant discrepancies between van’t Hoff and calorimetric enthalpies. III. Biophys. Chem. 64, 121–126. ArticlePubMedCASGoogle Scholar
- Tellinghuisen, J. (2005). Statistical error in isothermal titration calorimetry: variance function estimation from generalized least squares. Anal. Biochem. 343, 106–115. ArticlePubMedCASGoogle Scholar
- Lemay, J. F., and Lafontaine, D. A. (2007). Core requirements of the adenine riboswitch aptamer for ligand binding. RNA13, 339–350. ArticlePubMedCASGoogle Scholar
- Wadso, I., and Goldberg, R. N. (2001). Standards in isothermal titration calorimetry. Pure Appl. Chem. 73, 1625–1639. ArticleCASGoogle Scholar
- Tellinghuisen, J. (2007). Optimizing experimental parameters in isothermal titration calorimetry: variable volume procedures. J. Phys. Chem. B111, 11531–11537. ArticlePubMedCASGoogle Scholar
- Batey, R. T., and Kieft, J. S. (2007). Improved native affinity purification of RNA. RNA13, 1384–1389. ArticlePubMedCASGoogle Scholar
Acknowledgments
The authors would like to thank Deborah Wuttke and Jonas Fast for useful discussions on optimizing ITC experiments. This work was made possible by a Research Scholar Grant from the American Cancer Society to R.T.B.
Author information
Authors and Affiliations
- Department of Chemistry and Biochemistry, University of Colorado, Boulder, Campus Box 215, Boulder, CO 80309-0215, USA Sunny D. Gilbert & Robert T. Batey
- Sunny D. Gilbert